Introduction
Electrochemical reduction of CO_$2$ has attracted researchers’ attention as it has the potential to utilize the abundant greenhouse gas in the Earth’s atmosphere and store intermittent energy from solar panels and wind turbines in chemical bonds. A commercially viable catalyst for CO2 electroreduction should meet the cost-effective requirement while possessing high efficiency and selectivity. Experimental studies showed that Copper (Cu) can catalyze direct electrochemical reduction of CO2 to hydrocarbons in an aqueous bicarbonate solution with a high current density. Some Studies also showed there was a surface evolution of Cu surface under the reducing potential.
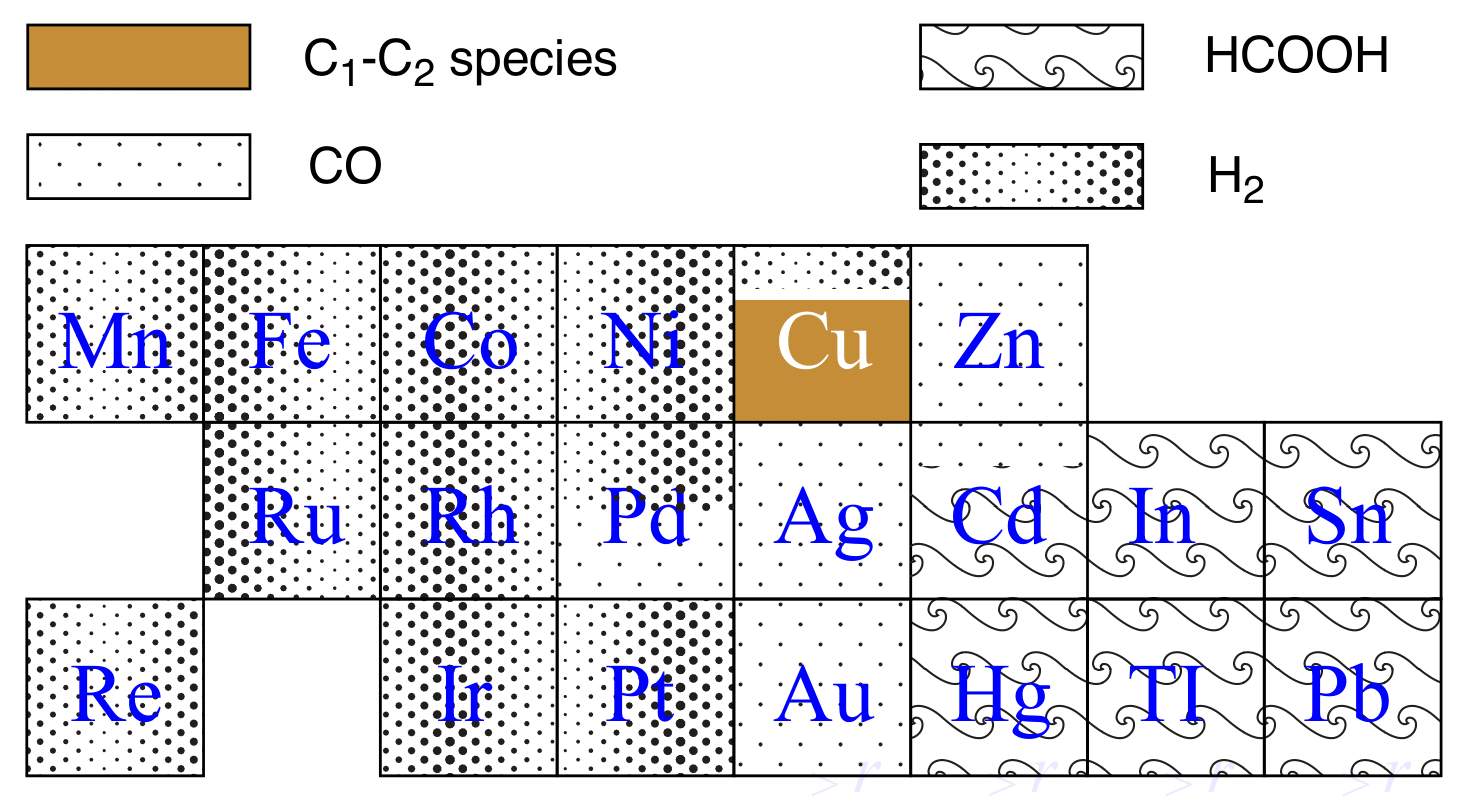
Challenges
- ... read more
Comments